By Jim Ross, President & CTO, Axion Biosystems
The ability to study human physiology often relies on biological models but recapitulating human development and disease comprehensively in a laboratory is a challenge. Most in vitro models rely on two-dimensional systems, which offer valuable insight into how cells function in healthy and diseased states but don’t allow scientists to extrapolate reliable information about how a whole organism works. Animal models offer a closer approximation of human anatomy and physiology, but they still don’t paint a complete picture. Since the popularization of stem cells in research, scientists have developed a new type of model that reflects many of the benefits of both cell and animal models: the three-dimensional organoid.
Organoids are derived from stem cells and self-organize to form complex, multicellular structures that mimic organ-level function. Scientists can generate them from various stem cell sources, such as embryonic stem cells (ESCs), induced pluripotent stem cells (iPSCs), or adult stem cells (ASCs). Scientists can collect or produce stem cells from patients with a specific disease and then use specific molecular cues to coax them into becoming a particular cell type or organ-like structure. This ability to directly recapitulate someone’s disease in a dish using a patient’s cells presents a notable advantage over animal models. As organoid research continues, scientists are finding that the organoid model provides an endless array of applications.
Tapping into the brain with organoids
Scientists can develop organoids that reflect virtually any organ, but one of the most studied and promising is the cerebral organoid, also referred to as a brain organoid, or “mini-brain.” The brain is unparalleled in its complexity and is linked to many poorly understood diseases. These characteristics have led to significant interest in studying this organ. Unfortunately, challenges unique to studying the brain can hinder research. For instance, obtaining living human samples is impractical, and recreating the brain’s multicellular composition, organization, and function is currently impossible. However, cerebral organoids are addressing this significant gap in neuroscience research.
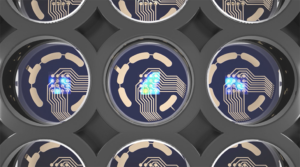
Multiwell solutions allow scientists to track up to 96 “brain-in-a-dish” cultures simultaneously, accelerating scientific discovery and changing the way scientists ask questions.
Researchers can develop brain organoids that replicate specific regions or developmental stages of this organ. So far, scientists have created cortical, hippocampal, thalamic, hypothalamic, midbrain, cerebellar, and retinal organoids (TANG). Brain organoids not only drive research on normal brain development across species (Fernandes), but these region-specific models also broaden their utility for studying neurodegenerative diseases in humans. For instance, midbrain organoids are perfect for studying Parkinson’s disease (TANG). On the other hand, cortical organoids are well-suited for Alzheimer’s disease research (Chen).
Additionally, researchers are developing assembloids — fusions of multiple organoids — to understand interactions between distinct brain regions. These fused structures are particularly relevant to studying the brain’s remarkably intricate neuronal interactions and circuit formation. One step beyond, researchers are creating connectoids, or organoids connected by axonal tracts. These more accurately model neural circuits (Chow).
Researchers study brain organoids so they can assess brain function. Analyzing neurons’ electrical activity can help scientists understand different brain regions and distinguish between abnormal and normal signaling. A standard tool for measuring the electrical activity of organoids is the bioelectronic assay. This assay consists of a multiwell plate containing multielectrode arrays at the bottom of each well that can detect the electrical activities of the cells above them. The bioelectronic assay is non-invasive, simple, and can measure network-level activity in real time. In one study, researchers sought to characterize a model of Alzheimer’s disease (AD) in which cortical organoids were exposed to a serum that helped them mimic the loss of the blood-brain barrier (Chen). A bioelectronic assay revealed reduced neuronal activity, a typical finding in AD.
Another study used a bioelectronic assay to assess neuronal activity in human forebrain organoids. These researchers explored the association between valproic acid (VPA) exposure and autism risk (Meng). VPA exposure disrupted synaptic transmission, reflecting findings in autism spectrum disorder. A bioelectronic assay allows researchers to compare in vitro electrical activity to in vivo human electroencephalography (EEG) recordings (Puppo). This ability will help researchers validate their in vitro models.
An organoid for nearly every organ
Within the last decade, the number of organoid models noted in the literature grew drastically, and new variations continue to emerge. Current organoid types include the intestine, kidney, liver, pancreas, lung, cartilage, bone marrow, dentin-pulp, skin, breast, heart, vascular networks, prostate, and others. Recent additions include three-dimensional models for the epididymis (Cyr) and the female reproductive tract (Venkata). Furthermore, older organoid models are also seeing improvements. A new generation of mini-hearts better resembles cardiac physiology, and scientists plan to use these organoids to study heart development and congenital diseases (Lewis–Israeli). Organoids are also an instrumental tool for studying infectious diseases. SARS-Cov-2 research uses a variety of organoid models as the virus causes symptoms across many parts of the body (Han). The field continues to advance as scientists attempt to create organoids consisting of multiple organ-like structures to study interactions between systems (Tang). Due to their wide-ranging applications, organoids are becoming ubiquitous.
Organoids are still in their infancy
As with two-dimensional models and animal models, organoids have their limitations. Organoids’ inherent versatility is also their greatest challenge. These three-dimensional models vary significantly, partly due to the different methods used to create them. In cerebral organoids, choosing one method over another can influence the structure and cellular composition (Atamian). Even when using the same method, inconsistencies are evident between batches. Separately, generating organoids is a lengthy and costly process. Organoids also lack vasculature; thus, organoids can only reach a certain size. Vascularization of organoids is critical for extending viability for further maturation, and it remains an active area of research (Zhang, Tang).
Further, organoid model development is outpacing the technology available for comprehensive analysis. Researchers successfully use existing resources, though there is much more to explore. For example, researchers are currently developing assays tailored for three-dimensional systems and biotechnology groups are redesigning MEA assays with organoids in mind. As new technology reaches the market, researchers will develop a deeper understanding of organoid maturation and characteristics, making it easier to standardize development and increase the model’s utility.
Organoids outside of the lab
Organoids have found use beyond basic research. Organoid biobanking is gaining traction, notably in cancer (Li). These biobanks have remarkable potential, given that the specimens are viable and closely recapitulate human organs. Patient-derived organoids (PDO) can help scientists determine the most effective therapy for an individual and advance efforts toward personalized medicine (Lucafo, Demyan). Numerous clinical studies assessing drug sensitivity and response in PDOs to guide therapy decisions are in motion.
Similarly, the ability of PDOs to predict drug response is relevant to clinical trial design. PDOs can serve as a screening tool to identify and group participants based on their expected responses (Bose). Likewise, organoids are bringing new possibilities to the regenerative medicine field. They also offer a new source for organ transplantation. Researchers are testing the feasibility of organoid transplantation in animals with successful outcomes (TANG). Finally, organoids can pair with existing technology like gene therapy. Scientists can generate organoids from genetically modified stem cells to model genetic diseases and mutations or repair genes in PDOs.
The organoid model is here to stay. Organoids allow researchers to approach human and disease research from a new perspective. Scientists can answer complex questions and stimulate new ideas with the help of organoids. These three-dimensional models are relevant to many applications, from biobanking to personalized medicine to organ transplantation. Organoids will continue to develop as the tools and technology for producing and analyzing these biological models adapt.
References
Tang XY, Wu S, Wang D, et al. Human organoids in basic research and clinical applications. Signal Transduct Target Ther. 2022;7(1):168. Published 2022 May 24. doi:10.1038/s41392-022-01024-9
Chen X, Sun G, Tian E, et al. Modeling Sporadic Alzheimer’s Disease in Human Brain Organoids under Serum Exposure. Adv Sci (Weinh). 2021;8(18):e2101462. doi:10.1002/advs.202101462
Chow SYA, Hu H, Osaki T, Levi T, Ikeuchi Y. Advances in construction and modeling of functional neural circuits in vitro [published online ahead of print, 2022 Aug 9]. Neurochem Res. 2022;10.1007/s11064-022-03682-1. doi:10.1007/s11064-022-03682-1
Fernandes S et al. Unraveling Human Brain Development and Evolution Using Organoid Models. Frontiers in Cell and Developmental Biology. 2021.
Meng Q, Zhang W, Wang X, et al. Human forebrain organoids reveal connections between valproic acid exposure and autism risk. Transl Psychiatry. 2022;12(1):130. Published 2022 Mar 29. doi:10.1038/s41398-022-01898-x
Puppo F, Muotri AR. Network and Microcircuitry Development in Human Brain Organoids [published online ahead of print, 2022 Aug 6]. Biol Psychiatry. 2022;S0006-3223(22)01437-8.
Cyr DG, Pinel L. Emerging organoid models to study the epididymis in male reproductive toxicology [published online ahead of print, 2022 Jul 8]. Reprod Toxicol. 2022;112:88-99.
Venkata VD, Jamaluddin MFB, Goad J, et al. Development and characterization of human fetal female reproductive tract organoids to understand Müllerian duct anomalies. Proc Natl Acad Sci U S A. 2022;119(30):e2118054119.
Lewis-Israeli, Y.R., Wasserman, A.H., Gabalski, M.A. et al. Self-assembling human heart organoids for the modeling of cardiac development and congenital heart disease. Nat Commun. 2021; 12, 5142
Han Y, Yang L, Lacko LA, Chen S. Human organoid models to study SARS-CoV-2 infection. Nat Methods. 2022;19(4):418-428. doi:10.1038/s41592-022-01453-y
Atamian, A., Birtele, M. & Quadrato, G. Not all cortical organoids are created equal. Nat Cell Biol 24, 805–806 (2022)
Zhang S, Kan EL, Kamm RD. Integrating functional vasculature into organoid culture: A biomechanical perspective. APL Bioeng. 2022;6(3):030401. Published 2022 Jul 6.
Li H, Liu H, Chen K. Living biobank-based cancer organoids: prospects and challenges in cancer research [published online ahead of print, 2022 Jul 21]. Cancer Biol Med. 2022;19(7):965-982.
Lucafò M, Muzzo A, et al. Patient-derived organoids for therapy personalization in inflammatory bowel diseases. World J Gastroenterol. 2022 Jun 28;28(24):2636-2653.
Demyan L, Habowski AN, Plenker D, et al. Pancreatic Cancer Patient-derived Organoids Can Predict Response to Neoadjuvant Chemotherapy. Ann Surg. 2022;276(3):450-462.
Bose S, Clevers H, Shen X. Promises and Challenges of Organoid-Guided Precision Medicine. Med (N Y). 2021;2(9):1011-1026. doi:10.1016/j.medj.2021.08.005
Tell Us What You Think!